Mechanism
What is a Mechanism?
Mechanisms define the functional relationships between cause and effect within and between levels of organism organization. Mechanisms of behavior work on various levels from microscopic (chemical signaling) to macroscopic ( social interaction). The mechanisms which produces mimicry behavior in Cephalopods can be studied mechanistically on various levels.
• Cephalopods exhibit primarily defensive mimicry using background textures, environmental objects and other animals as models.
• Changes in color are used to mimic backgrounds and environmental objects. These changes are produced by chromatophores, reflector cells, and leucophores, but the concentration of chromatophores varies across species.
• Changes in body shape depend upon hydrostatic changes in the musculature. A lot of research has been done on arm movements, changes in body shape and gait in the cephalopds.
• In order to form a proper disguise a fine tuned visual system and complex neural circuitry work together.
Defensive Mimicry
The most important defense mechanism of most cephalopods is their ability to alter their color, shape and texture in order to blend in with their surroundings . They can create a multitude of disruptive patterns that aid in their camouflage. These patterns allow cephalopods to generate visual signals for defense. Cephalopods can flash different patterns in rapid succession in order to confuse potential predators.(Hanlon and Messenger, 1996)
Chromatophores
Cephalopods are able to change their color as a result of color cells called chromatophores. The colors they produce range from reddish brown to yellow-orange. They contain pigment granules and are surrounded by radial muscles. With the contraction and expansion of these muscles, the pigmentation of the chromatophores changes. These muscles are independently controlled by the central nervous system. While some chromatophores expand others may contract. This allows cephalopods to change color almost instantaneously. (Hanlon and Messenger, 1996).
The chromatophores are complemented by reflecting cells. These cells produce various colors by refracting light, and white by reflecting light. There are three types of reflecting cells.
Iridophores reflect mainly pinks, yellows, greens, blues and silvers (Mathger, 2007).
Reflector cells, which are known only in octopuses, reflect blues and greens, and produce these colors by interference or diffraction.
Leucophores are broad-band reflectors, and they reflect white light or the wavelength that is most prevalent in the environment (Cousteau and Diolé, 1973; Hanlon and Messenger, 1996). The way octopuses gather information about their surroundings and use it to change color is not well understood. For instance, most scientists believe octopuses are color-blind (Hanlon and Messenger, 1996). Thus they cannot see the colors they are mimicking. Also, recently deceased octopuses can change their color according to their environment (Cousteau and Diolé, 1973) .
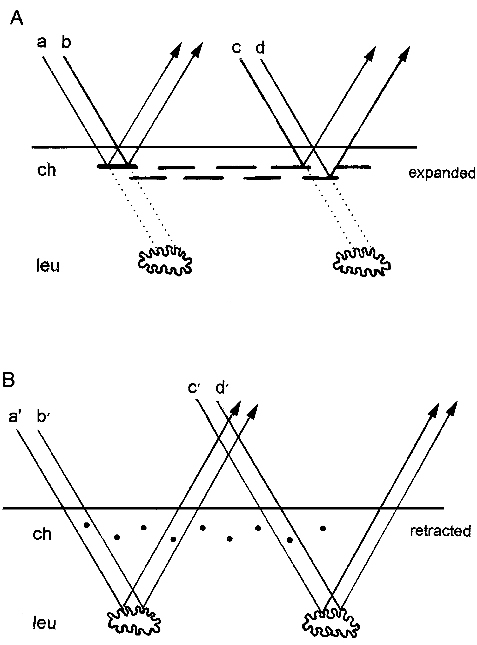
Role of leucophores in color matching in Octopus vulgaris. A. In dim light chromatophores (ch) expand and act a neutral density screen to match the brightness of the background(a-d) ambient light rays do not reach the deeper-lying leucophores (leu).B. In bright light, the chromatophores retract (to maintain the brightness match), allowing the ambient light to reach toe leucophores (a'-d'):which allows for accurate reflection of spectral characteristics.(Messenger, 2001)
Time lapse image of chromatophore change displaying the rapid color transformation . (Hanlon, 2007)
Cuttlefishes are able to change color and reproduce remarkably complex patterns on their skin almost instantaneously. According to Cousteau and Diolé (1973), when cuttlefishes were placed on top of plates with geometric designs such as straight lines, lozenges, or check patterns they were able to accurately reproduce multiple patterns. In cuttlefishes, a full cycle of expansion and contraction of the chromatophores takes about two-thirds of a second.
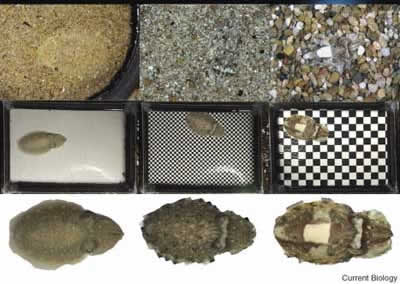
Responses of Cuttlefish in different mediums. The type of medium produces a distinct behavioral response from the cuttlefish. Cuttlefish display the unique ability to match varying granularities of surfaces.(Hanlon, 2007).
Arm and Body Movements
In order to create goal-directed arm or body movement, the nervous system must generate a sequence of motor commands that bring the arm toward the target or orchestrates the body to move in a uniform manner. Control of the octopus arm is especially complex because the arm can be moved in any direction, as it has nearly infinite degrees of freedom.The motor program which controls this voluntary movement is embedded within the neural circuitry of the arm itself.
There are four basic forms of movement demonstrated by octopi:
Jetting
The octopus expels water from the mantle through the funnel, which is pointed away from the posterior mantle to propel the animal backward. The body and arms trail behind the mantle and do not bear weight Arms are held tightly together.
Swimming
The octopus expels water from the mantle through the funnel, which is pointed backward toward the posterior mantle to propel the animal forward. Swimming may or may not incorporate undulations of the body and arms. While swimming no portion of the body rests on the bottom (see video).
http://www.journals.royalsoc.ac.uk/content/pp95mdq58uyhe4ru/media1.mov
Crawling
The octopus uses the sucker edge of more than two of eight arms to push and pull along the bottom of the ocean. Each arm typically contacts the bottom at multiple points. In general, octopuses crawl by pushing and pulling themselves along the bottom using what appear to be irregular (Mather, 1988) and intermittent movements using multiple arms. In at least one species of octopus though there are joint like points along the arm where oposing muscle groups meet (Huffard 2006) (see video for crawling octopus).
http://www.journals.royalsoc.ac.uk/content/pp95mdq58uyhe4ru/media3.mov
Bipedal Walking or Running
The octopus pushes or rolls along the bottom using alternating movements of a single pair of arms (see video).
http://www.sciencemag.org/content/vol307/issue5717/images/data/1927/DC1/1109616s1.mov
Crawling is the most common form of locomotion for octopuses in the wild when moving at relatively slow paces during activities away from the den. Fast transport typically involves jet propulsion, in which octopuses bring water into their cavity and then quickly expel it . Although it is the main form of fast escape, jet propulsion is physiologically inefficient, quickly leading to oxygen debt and also requiring internal mantle pressures which are physiologically dangerous (Wells, 1990, Huffard 2006). Bipedal locomotion provides some octopuses with another means to move faster than crawling during threatening situations, while freeing six arms for use in cryptic postures (Huffard 2005).
Gait
There have been two octopuses that can walk using two legs. Octopus marginatus and Octopus (Abdopus) aculeatus move along sand using two legs in a rolling gait. This locomotion differs from normal crawling, which involves several arms sprawling around the body and the suckers to push and pull the animal along. When walking Octopus marginatus leans back on its ventral most arm pair and uses these back appendages to propel itself. Each leg is usually on the ground more than 50% of the time which qualifies the behavior as walking as opposed to running. The Octopus aculeatus incorporates its walking into a cryptic display designed to mimic algae. It sticks its legs in the air and rolls back on its rear legs. Each arm contacts the ground with at least 75% of its length, creating a conveyor belt along which the octopus moves. (Huffard 2005).
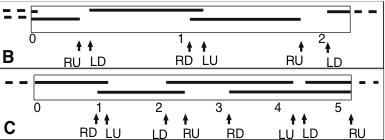
Analysis of left and right arm - ground contact for B) O. Marginatus and C) O. Aculeatus (Huffard 2005). R and L stand for right and left arms while U and D stand for up and down respectively.
Transverse, longitudinal, and oblique bands of muscle in the arms create flexible and internally stable structure that supports the octopi while they walk. Contractions of muscles spread down the arms from base to tip in a stereotypical manner. This motion can be triggered using circuitry in the arm itself indicating that the requirement for bipedal motion is not entirely dependent upon the brain for its generation (Huffard 2005).
Complex Neural Circuitry
Tublitz et al (2006) showed that chromatophore muscles are regulated by two different excitatory transmitters, glutamate and the family of FMRFamide-related peptides (FaRPs). Glutamate mediates rapid and transient chromatophore cell expansion whereas the FaRPs are responsible for slower, more sustained responses. Messenger (2001) lists many more neurotransmitters in the system controling mimicry including acetylcholine, dopamine, nonadrenaline, serotonin, GABA, L-glutamate, and octopamine. In Octopus vulgaris there are five lobes of the brain and over 600,000 nerve cells that regulate the activity of the chromatophore system. These five lobes are capable of producing the complex behavior observed but need input from many other parts of the cephalopod brain to produce convincing mimicry. The details of this system are not yet fully understood.
Visual Processing
Cephalopods are very good at detecting edges, contrasts, area of objects, and density of white objects on black backgrounds. While they are colorblind this does not seem to interfere with their ability to mimic the environment around them. Cuttlefish have also been shown to use their own size as a factor in the generation of disruptive coloration (Barbosa, 2007).
|